This article discusses the dynamics of the development of a free turbulent jet emanating from a square nozzle of the exhaust cut. The studies include cases where external impact on the flow was and was not eliminated. The hot-wire anemometer was used to measure the average and flactuation velocity values, and analyze the turbulence levels. Fluctuations in the temperature of free jetnondimentional overstress propagating from the nozzle are investigated.
Key words: nozzle, free flow, turbulent flow, experimental study, velocity and temperature profiles.
В данной статье приведены результаты исследования динамики развития свободной турбулентной струи, вытекающей из сопла с квадратным выходным сечением. Исследования проводились как при наличии внешнего воздействия, так и без воздействия. Средние и пульсационные значения скорости измерялись с использованием термоанемометра, был проведен анализ уровня турбулентности. Исследовались безразмерные профили осевой скорости и получена формула, которая описывает изменение данной скорости. Исследовались безразмерные поперечные профили скорости и температуры свободной струи.
Ключевые слова: сопло, свободная струя, турбулентное течение, экспериментальное исследование, профили температуры и скорости.
.
Free turbulent jet flowing from a square-shape nozzle of the exhaust cross-section has not yet been studied in a wide range of velocities to sound speed. If two opposite sides of the square-shaped outlet section are kept motionless and two sides are stretched perpendicular to them, then we get a rectangular nozzle (the determining parameter is the stretching parameter λ, where λ=а/b , a is the length of the outlet section; b is the width of the outlet section). The flow coming from such a nozzle is three-dimensional. Such three-dimensional turbulent free jets have been studied in general abundance [1–12]. And if we take a flow that is likely to propagate from a square cross-section nozzle (λ = 1), we can only note some insignificant works covering this subject [5; 10; 14; 15]. With regard to the investigational study of a turbulent jet outgoing from a square outlet section nozzle, one can mention the work [16]. In this paper, the average-minute characteristics of a turbulent free air jet escaping from the nozzle are widely investigated. The study was also carried out in cases where the external(acoustic) impact on the flow was or was not eliminated.
This paper presents the results of further investigation of such a jet.
Investigational study equipment
The experiment was carried out using the probe shown in Figure 1.
The air (2) coming out of the fan (1) enters from the vibration-absorbing wire (3) into the quiescent chamber, and then flows out of the nozzle with a square shape of the output image (6) through the gratings (4) and (5).
The main part of the flow is located in the slurry part of the ИАБ-451 shadow instrument. It can be used to explore the shadow snapshot. The impact on the flow was carried out using dynamik (7) with a power of scales 50Wt, frontally located on the output image of the flow in the quiescent chamber.
A sinusoidal signal is supplied from the sound generator (13) to the speaker, in connection with which a sinusoidal oscillation of velocity at the selected frequency occurs on a given sound image.
A Pito tube and an MMН-240 micromanometer (9) were microlated for a moderately fast and dynamic gate measurement.
A thermoanemometric blocks system was used in the work. It consists of a two-channel thermoanemometric system with a linear output signal velocity, a temperature transducer, and a phase selection unit (12).
The position of the Pito tube and transducer along the three symmetricaxes is carried out using a three-dimensional coordinate.
The design of a square-shaped nozzle with a profiled section using the Vitoshinsky formula is shown in Fig. 2 (in addition to the figure, the coordinate axes have also been plotted). Nozzle compression rate
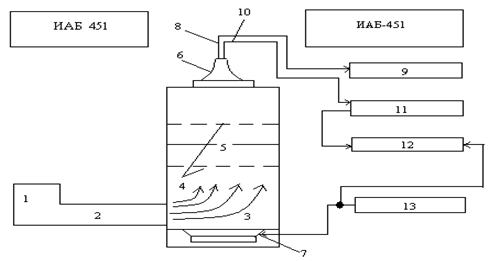
Fig. 1. Experimental Set-Up Diagram: 1-fan; 2-vibration-absorbing wire; 3-chamber of rest; 4-control grids; 5-heating grate; 6-nozzle; 7-speaker; 8-Pito tube; 9-micromanometer; 10-sensor; 11- СТМ-02 type thermoanemometric system block; 12-phase selection block; 13-sound generator
Fig. 2. Square nozzle design: 1-nozzle wall; 2-nozzle support
Findings and Analysis
To study the vortex structure of the flow in the flow, we used the method of recording the instantaneous velocity pulsation measured by a thermoanemometer, which is saved to the oscilloscope screen.
The thermoanemometer was used to measure average and pulsation velocity values, and the levels of turbulence (turbulence intensity). Based on the oscillograms analysis of instantaneous change in the velocity pulsation, the development frequencies were calculated when primary vortices appeared and moved away from the nozzle.
Oscillograph record analysis of the stretching waveform of the instantaneous velocity ripple shows that velocity fluctuations have well-defined frequencies. These velocity fluctuations exactly coincide with the frequencies of discrete vortices formation in the free boundary layer in the initial compartment. As the distance downstream from the outlet section of the low nozzle increases, as a result of interaction of the first-order vortices with each other and their enlargement, on this basis, low-frequency velocity oscillations with large amplitude arise.
In addition, high-frequency velocity pulsation persists against their background, which is explained by primary vortices. As an example, Fig. 3 shows oscillograms of the flow rate fluctuations from a nozzle with a square outlet section, obtained at different distances along the flow axis, at different values of the х/b parameter (caliber).
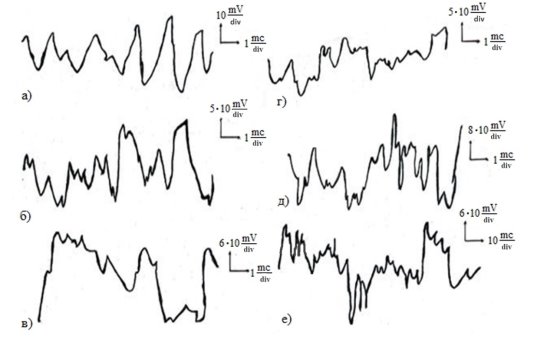
Fig.3. Oscillograms of velocity pulsations along the axis of the flow propagating from a nozzle with a square outlet section:
The study showed that due to a decrease in the average speed, the number of vortices passing by the sensor per unit of time decreases. The conclusion from these findings is that with distance from the nozzle downstream, the vortices do not split into smaller vortices, the number of vortices does not increase, but rather they merger into larger vortices of large sizes. On the other hand, each vortex within these groups can retain its individuality at very large distances from the nozzle outlet (up to 30 gauges) and cause velocity pulsation.
The work also investigates the free flowpulsation characteristics. The thermoanemometer was calibrated before measurements were taken. The pulsation intensity (intensity of turbulence)
Where,
Regularities of turbulence intensity distribution along the axis at the square nozzle of the exit section are shown in Figures 4 and 5. As can be seen from the images, in both cases (whether or not there is an effect)
Fig. 4. Axial distribution of turbulence intensity in the flow:
The paper investigates the velocity profiles in the free jet cross-sections outgoing from the square-shaped nozzle of the outlet section. The experimental findingsare shown in Fig. 6. The experiment was performed when eliminating the external impact on the flow, the frequency of exposure
Fig.5. Increasing distribution of turbulence intensity in the flow:
Fig.6. Velocity profiles in cross-sections of free flow outgoing from an outlet section square nozzle:
Fig. 7 shows the changes in the axial velocities of free turbulent jets flowing from the square and circular (axisymmetric) nozzle of the outlet section. The initial current velocity is about 21 m/s. The test results correspond to a situation where the flow was not affected. As can be seen from the figure, the change in axial velocity in both cases occurs according to the same pattern. The third continuous line in the figure is a theoretical straight line drawn according to the following expression (formula), obtained in theory from our research:
As can be seen, the theoretical straightness is in good alignment with the experimental results. The coincidence rate of the obtained formula with the theoretical expressions [3; 13], reflecting the change in the axial velocity in the main section of the axisymmetric flow, is at a high level.
Fig. 7. Axial velocity change:
Some technical challenges were encountered in obtaining a uniform temperature profile at the jet section outlet. They were eliminated, first of all, because tiny cellular nickel grids were selected, and secondly, because the terminals were located close to each other.
In this regard, the temperature profile at the nozzle outlet can be considered uniform. Slight deviations were only observed in areas where heat from the inner sides of the nozzle spreads outward. Therefore, to minimize the deviation of the transverse temperature profile at the nozzle outlet from the rectangular shape, the inner sides of the nozzle were thermally insulated from the external environment.
The paper shows the dimensionless profiles of the average temperature in the main section of the buoyant jet from asquare shape nozzle of the outlet section, of various cross sections (Fig. 8).
Fig. 8. Temperature fluctuations of the dimensionless overvoltage of the free jet propagating from the square-shape nozzle of the outlet section along the cross section.U=21м/с;f =0;
As the figure shows, the versatility of cross-sectional temperature profiles starts at the beginning and continues to the end of the main compartment.
References:
- Trentacoste N., Sforza P. M. Further experimental results for three-dimensional free jets. AIAA J. 1967.Vol.5. No.5. Pp. 885–890.
- Sfeir A. A. The velocity and temperature fields of rectangular jets. Int. J.Heat and Mass Transfer. 1976. Vol.19. No.11. Pp 1289–1297.
- Крашенинников С. Ю. Рогальская Е. Г. Распространение струй из прямоугольных сопел, свободных и вблизи экрана. Изв. АН СССР. МЖГ. 1979. № 4. С. 39–48.
- Абрамович Г. Н. О деформации поперечного сечения прямоугольной турбулентной струи. Изв. АНСССР. МЖГ. 1983. № 1. С.54–63.
- Quinn W. R. Turbulent free jet flows issuind from sharp-edged rectangular slots: the influence of slot aspect ratio. Exp. Thermal Fluid Sci. 1992. Vol.5. No.2. Pp.203–215.
- Wilson R. V., Demuren A. O. Numerical simulation of turbulent jets with rectangular cross-section. ASME FED. 1996. No.238. Pp. 121–127.
- Holdo A. E., Simpson B. A. F. Simulation of high-aspect-ratio jets. Int. J. Numer. Methods Fluids. 2002. Vol.39. No.4. Pp. 343–359.
- Rembold B., Adams N. A., Kleiser L. Direct numerical simulation of a transitional rectangular jets. Int. J. Heat Fluid Flow. 2002. Vol.23. Issue 5. Pp. 547–553.
- Berg J. R., Ormiston S. J., Soliman H. M. Prediction of the flow structure in a turbulent rectangular free jet. Int. Commun. Heat and Mass Transf. 2006. Vol.33. No.5. Pp. 552–563.
- Faghani E., Maddahian R., Faghani P., and Farhanieh B. Numerical investigation of turbulent free jet flows issuind from rectangular nozzles: the influence of small aspect ratio. Archive of applied mechanics. 2010. Vol. 80. No.7. Pp. 727- 745.
- Исатаев С. И., Толеуов Г., Исатаев М. С., Болысбекова Ш. А. Экспериментальнoе исследованиe трехмерных турбулентных струй, истекающих из сопла с прямоугольным выходным сечением. Инженерно-физический журнал. 2016. Т.89, № 2. С. 383– 387.
- Исатаев М. С., Толеуов Г., Есеналина К. А. Экспериментальное исследование распростронения трехмерных турбулентных струй из прямоугольных сопел. Инженерно-физический журнал. 2017. -Т.90, -№ 6. -С.1543–1548.
- Aбpaмoвич Г. Н., Гиpшoвич Т. A., Кpaшeнинникoв C. Ю. и дp. Тeopия туpбулeнтныxcтpуи. Изд. 2-oe пepepaб. и дoп. Пoдpeд. Г. Н. Aбpaмoвичa. — М., 1984. -720 c.
- Tsuchiya Y., Horikoshi C., Sato T.On the spread of rectangular jets. Exp. Fluids 4. 1986. -Р.197–204.
- Quinn W. R., Militzer J. Experimental and numerical study of a turbulent free square jet. Phys. Fluids 31. 1988. -Р.1017–1025.
- Төлеуов Ғ., Исатаев М. С., Оралбаев А. Б., Артықбаева А., Алтайқызы М., Асильбекова Ш.Шығар қимасы квадрат формалы соплодан ағып шығатын еркін турбулентті ағыншаны эксперименттік зерттеу // ҚазҰТЗУ хабаршысы. — № 2(126). Алматы, 2018.-Б.233–242.