New representatives of polyoxometalates containing rare earth elements derivatives of polyoxophosphomolybdic acid (GPFM) and polyoxotungstate supported on aluminum oxide have been synthesized. The structure of the REE-POM samples were characterized by X-ray phase analysis, IR Fourier spectroscopy, electron paramagnetic resonance and scanning electron microscopy. These catalysts are highly active in the hydrogenation of petroleum fractions processes.
Keywords: polyoxomolibdate, polyoxotungstate, petroleum fractions, IR-spectroscopy, EPR
Environmental requirements for the quality of hydrocarbon bases of fuels and oils are becoming more stringent, which implies a reduced content of sulfur compounds and aromatic hydrocarbons in their composition. The change in the chemical composition of petroleum fractions occurs as a result of the reactions of hydrogenolysis of sulfur- and nitrogen-containing compounds, hydrogenation of olefins, arenes and resins in the process of hydrotreatment in the presence of sulfide catalysts based on Mo, Co. In the production of fuels, mixtures of straight-run petroleum fractions with fractions of thermal catalytic (destructive) processes are subjected to hydrotreating. In these fractions, there are much more sulfur- and nitrogen-containing compounds, olefins and polycyclic arenes that are stable under hydrotreatment conditions, than in straight-run petroleum fractions. Thus, a comprehensive study of the physicochemical characteristics of petroleum fractions used for the synthesis of motor fuels should be considered relevant [1–3].
Catalysts are a key factor in the hydrotreating process. One of the ways to increase the activity of catalysts and create catalytic compositions for the hydrotreatment of specific petroleum fractions is the introduction of modifying additives. However, existing methods for introducing these additives often lead to the formation of a complex mixture of oxide phases of indefinite composition [4]. This does not allow the directed synthesis of an oxide precursor of a sulfide catalyst whose active phase includes the main active component (Mo or W), a promoter (REM), and a modifier. Thus, studies aimed at finding methods for the synthesis of oxide precursors of hydrotreating catalysts with an active phase of a given composition are topical [5].
The purpose of this work is to study the regularities of the conversion of sulfur compounds and unsaturated hydrocarbons of petroleum fractions in the presence of catalysts based on POM.
The choice of the composition of catalytic complexes for the hydrotreatment of various oil fractions involves the study of the regularities in the course of the main reactions of the hydrotreatment of oil fractions at elevated pressure. Currently, tungsten-containing catalysts are of particular interest because of their high activity in hydrogenation processes and stability at elevated temperatures. However, hydrotreatment catalysts, in which tungsten rather than molybdenum is the main active element, have not been widely used in industrial practice due to the difficulty of converting WO 3 into systems. Therefore, a mixed system was chosen — POM promoted applied to A1 2 0 3 [6–7].
Continuing to study the structure of the synthesized catalysts, IR spectra were taken at selected points on the surface of the catalyst under a microscope with the LUMOS IR-Fourier device. For this purpose, polyoxomolobdate modified with Gd, Nd, La, Pr and their peroxoforms were analyzed. As an example, consider a modified peroxomolybdate catalyst with Gd. This catalyst was first microphotographed under a microscope, then 5 points were selected on the surface of the catalyst in the obtained microphotography and IR spectra were drawn for each point.
At point 1 of IR spectra, O — Mo — O — Pr, O — Mo — O and correlated absorption bands corresponding to 829–868 cm -1 , 922 cm -1 are observed. There are also absorption bands in the spectrum corresponding to the deformation (1100 cm -1 ) and valence oscillations (3303, 3597 cm -1 ) of the O — H bond (Figure 1 a). 1042, 1089 cm -1 are absorption bands corresponding to the P — O — bond. At the same time, 986 cm -1 and 690 cm -1 absorption bands are present in the spectrum, respectively, according to — O — O– and Mo — O — O bonds. Comparison of the IR spectra of points 1 and 2, 3, 4, 5 shows that they are almost identical. This indicates that the catalyst is homogeneous. A microscopic view of the catalyst surface and a comparative description of the points are given in Figure 1. (a) and Figure 1. (b)
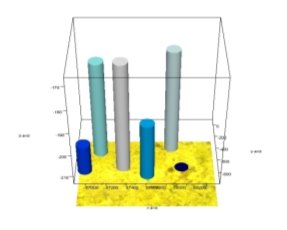
(a) (b)
Figure 1. Microphotography of the catalyst surface (a), its 3D image (b)
However, the IR spectra of the Nd-modified and oxalate-treated polyoxomolybdate and microscopically selected points under the microscope are slightly different from the samples of the gadolinium polyoxomolybdate catalyst studied with the LUMOS IR-Fourier spectrometer. It should be noted that these samples are more effective in the oxidation reactions of olefins than Gd-modified samples.
Six points were selected on the surface of the catalyst and their IR spectra were plotted. In contrast to the spectrum of Gd drawn in the same way, an absorption band of 1868 cm -1 corresponding to the — O — O — CO group is observed. Comparison of points shows that points 1, 2, 5, 6 on the surface are identical to each other, and points 3 and 4 are identical to each other.
Fig. 2. Microphotography of the catalyst surface (a), its 3D image (b)
Fig. 3. IR spectra of selected points on the surface of Nd-containing polyoxomolybdate catalyst
The surface morphology of polyoxomolybdate with Nd-containing -Nd n PMo m O 40 (Gd n PW m O 40 ) (where n = 3–6, m = 12–14) is characterized by a surface consisting of 1–2 μm fragments. In this case, in contrast to the primary polyoxomolybdate complex, partial amorphization and increase in the dispersion of the crystal structure are observed in the peroxocomplex. The decomposition of the structure of the primary polyoxomolybdate complex when mixed with a solution of hydrogen peroxide is also confirmed in the X-ray phase analysis of their individual samples. Figure 4 describes the mapping of the presented catalyst under a microscope.
Fig. 4. Microphotography of NdPO 4 ∙H 2 O∙MoO 2 P 0,5 Mo 14 O 42 system polyoxomolybdate complex
A higher result was obtained in the presence of Al 2 O 3 carbon material containing 15.0 % Mo n+ . Above it, the activity of samples containing Mo n+ does not change practically. The study of the stability of the catalyst selected on the basis of over time shows that it is very different from HNa-mordenite. Thus, the yield of spiroacetal in the presence of gadolinium-polyoxomolybdate catalyst prepared on Al 2 O 3 does not change practically during 7 experiments (Figure 5).
Taking into account these results, subsequent studies used catalyst samples impregnated on Al 2 O 3 containing 15.0 % Mo n+ .
Fig. 5. Change of NdPO 4 (MoO 2 ) 0,5 PMo 14 O 42 /Al 2 O 3 system (Mo n+ -15 %) stability
In order to determine the degree of hydrogenolysis of sulfur compounds and hydrogenation of PAH at elevated hydrogen pressure in the composition of light coking gas oil with a sulfur content of 2.06 wt. %, PAH — 14.32 wt. % catalyst Nd-POM/А1203 synthesized by use of HPC of 12 series: PMo 12 , PNdMo 11 , NdMo 12 was used. A series of MoO 3 /Al 2 O 3 catalysts with additions of tungsten from various compounds was also prepared: a mixed system — MoO 3 +\Al 2 O 3 promoted by Nd on Al 2 O 3 . The reference sample was a catalyst prepared on the basis of ammonium para molubdate and not containing WO 3 . All catalysts were synthesized by successive impregnation of the support with the Mo(W) compound and Nd nitrate with intermediate drying and calcination. Samples of Nd-polyoxomolybdate/Al 2 O 3 catalysts differ only in the presence of a heteroatom in the composition of the molybdenum compound. Samples of Nd-W(A1 2 O 3 + MoO 3 ) catalysts differ in the presence or absence of a heteroatom in the composition of the tungsten compound.
The sequence of the degree of hydrogenolysis of sulfur-containing compounds depending on the POM of molybdenum, on the basis of which the catalyst was prepared, differs from the sequence obtained in the conversion of thiophene in the presence of POM/Al 2 O 3 catalysts prepared by co-impregnation. In the hydrotreatment of light coking gas oil, the most significant increase in the degree of hydrogenolysis of sulfur compounds is observed in the case of using HPC catalysts containing Nd either as a complexing agent or in the coordination sphere for the synthesis.
The degree of PAH hydrogenation, in contrast to the degree of HDS of sulfur compounds, increases when POM catalysts are used for the synthesis. The maximum effect takes place, as in the case of the degree of HDS, for samples based on NdMo 12 . The degree of PAR hydrogenation in the presence of a catalyst based on NdMo 12 -POM at 340–390°C is 1.5–2 times higher than in the reference sample. The degree of hydrogenolysis of sulfur compounds and hydrogenation of PAH in the light thermal cracking gas oil composition is higher in the presence of POM(W)/(Al 2 O 3 + MoO 3 ) catalysts compared to the Mo/Al 2 O 3 catalyst.
Polycyclic arenes, along with olefins, are coke. An increased degree of PAH hydrogenation in the process of oil fraction hydrotreatment reduces coke deposits on the catalyst surface. Catalysts with a higher hydrogenation activity contain noticeably less coke than the reference catalyst. The content of sulfide sulfur on these catalysts is higher than on the reference sample.
Conclusion
It has been shown that an increase in the degree of POM of sulfur-containing compounds is accompanied by an increase in the degree of PAH hydrogenation, although the activity series of catalysts based on heteropoly compounds in relation to these reactions may not coincide. The high degree of PAH hydrogenation contributes to the reduction of coke deposits on the surface of catalysts based on POM. There is a correlation between a high degree of hydrogenolysis of sulfur-containing compounds and hydrogenation of PAH, a high degree of sulfidation of active components during catalyst activation, and a low coke content after testing.
References:
- Kostenko A. V., Ferkel E. V. The development of new catalysts — a strategic direction in the development of domestic oil refining.// Oil refining and petrochemistry. 2004. No. 4. P.43–46.
- T. A. Fedushchak, T. V. Petrenko, A. V. Vosmerikov, D. A. Kanashevich, S. P. Zhuravkov, L. M. Velichkina. Physicochemical properties and activity of nano powder catalysts in the hydrodesulfurization of diesel fraction, Russian Journal of Physical Chemistry A volume 86, pages 375–379 (2012)
- Pleshakova N. A., Shabalina T. N., Sheikina N. A., Shabalina E. O., Elasheva O. M., Tyshchenko V. A. // Science and technology in industry. No. 3–4. 2004. S. 28–31.
- Tomina N. N., Nikulshin P. A., Pimerzin A. A. Influence of Anderson- and Keggin-type heteropolycompounds as oxide phases precursors of hydrotreating catalysts on their activity // 14th ICC, Seoul, Korea, 2008. PI-41–05.
- Ganiyu, S. A. Synergy effect of boron and cobalt in B 2 O 3 -SBA-15-(Co)Mo catalyst for efficient hydrodesulfurization of liquid fuels. Res Chem Intermed 47, 3751–3768 (2021).
- Monteverdi S., Bettahar M. M., Begin D., Маgesye. Characteristics and hydrogenating properties of active carbon supported p-Mo2C // Fuel Processing Technology 77–78 (2002) 119–124.
- Phillips D. C., Sawhill S. I., Self R., Bussell M. E. Synthesis, characterization, and hydrodesulfurization properties of silica-supported molybdenum phosphide catalysts //Journal of Catalysis 207 (2) (2002) 266–273.